Note: This is the second article in the three-article series on particle and fundamental physics. Here are the first and third articles.
In this part of the article series, we’ll see the fundamental particles that comprise the Standard Model of particle physics, along with how they interact.
Index
Introduction
The Standard Model explains all phenomena of the Universe in terms of 61 particles, antiparticles included, and their interactions. The basic premise is that there are two kinds of particles in this universe: matter particles and mediating particles, called fermions and gauge bosons respectively.
The interactions are simply forces between all particles (fermions and bosons) and are mediated by gauge bosons. These interactions are fundamentally four. Each depends on a specific property of the particles it is acting on (mass or charge, for example). Every fundamental force has associated gauge bosons.

The Four Forces and Associated Gauge Bosons
The strong force
This force has the greatest magnitude but is extremely close range. Its study falls under quantum chromodynamics, QCD for short. The associated property for it is “color charge” (or simply colour) and is of six types – named red, green, blue, antired, antigreen and antiblue for ease of visualization. It has no relation to the actual colour we observe in daily life. The last three colour charge types are observed in antimatter.
A property of nature is that no isolated particle can have colour charge on it. In other words, all freely found particles must be colourless, with the colour charge combining in such a fashion that the net result is zero. One way to achieve this is by having equal amounts of red, green and blue colour charge. Another way is to have equal amounts of a specific colour and its anticolour (say, red and antired). These two kinds of combinations will come in handy later, as we shall see.
It is mediated by the gauge bosons called “gluons”, eight in number. All these fundamental particles have net colour charge and hence cannot be found in a free state. In fact, they are all found inside larger particles called baryons and mesons, which we shall see in the fermion section.
Since gluons have colour charge, they not only mediate the strong force but also experience it.
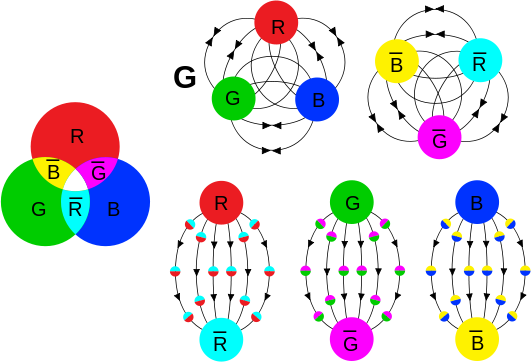
The electromagnetic force
This is the second strongest force and can act between particles very far apart. The associated property, in this case, is “electric charge”, and is of only two types: positive and negative. Its study falls under electrodynamics (or quantum electrodynamics on the particle scale).
Unlike in the case of colour charge, there is no property of nature that free particles cannot have net electric charge. In fact, the popular electron and proton, which can be made to exist in isolation, carry electric charge on them.
The gauge bosons in this interaction are photons, the very same particles that constitute visible light and the rest of the electromagnetic spectrum. Hence, photons are found everywhere, and, in the form of visible light, are the reason why we see things. Photons themselves are neutrally charged, and hence do not experience electromagnetic force.
All the contact forces we see in our daily life are merely manifestations of the electromagnetic force – the repulsions between electrons outside an atom is what makes the contact force exist.
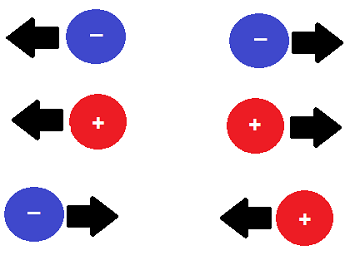
The weak force
This force is much weaker than the previous two and is observed in radioactive decay. The associated property is the funnily named “flavour” and comes in many types, which shall be discussed in the fermion section. In simple terms, flavour is an inherent property of particles, just like mass, and is expressed in terms of flavour quantum numbers. Interestingly, the work of Glashow, Salam and Weinberg in the 1960s showed that the weak force and electromagnetic force were both manifestations of a single electroweak force which occurred at high energies.
The mediating particles, in this case, are the intermediate vector bosons, named the W+, W- and the Z boson. All are extremely massive and extremely short-lived. They two W bosons are observed during radioactive decay and transmutation (change of one element to another), while the Z bosons are seen during the scattering of neutrinos.

The gravitational force
This is the weakest of all the fundamental forces. Its associated property is mass, which is of only one type – positive. Its study is a part of General Relativity.
It might seem surprising that this very noticeable force in daily life is also the weakest. This, however, has to do with the nature of matter. Most matter is electrically neutral and hence does not show dramatic electromagnetic interaction. No free matter has color charge; hence we do not observe the strong interaction either. And finally, the weak interaction is limited to nuclear processes, again something we do not see on a daily basis.
The particle which mediates gravitation is the graviton, which, however, has not been discovered. A reason for this is the extremely weak nature of gravity. However, the theoretical reasons are compelling to seriously consider the existence of this particle.
Now we have seen all the fundamental forces, but one boson is not yet introduced. This is the Higgs boson, the elusive particle whose existence was confirmed as recently as 2012. It is the reason why all massive particles have masses. It is not a gauge boson, like the force mediators discussed so far, but is a different kind called a scalar boson.
Fermions
All matter consists of fermions, which are primarily of two types: leptons and quarks.
Leptons
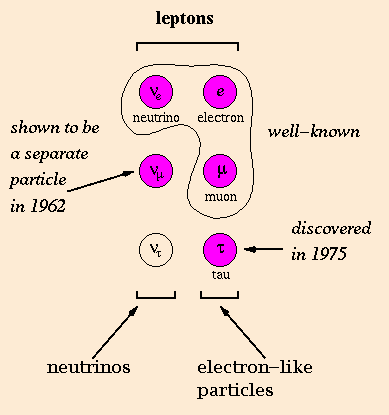
Leptons were initially considered to be a category of light mass particles. Later, thorough research showed that the absence of colour charge was the defining property of a lepton. Since they lack colour charge, leptons cannot interact via the strong force. However, they do possess mass, electric charge, and flavour, and hence interact via the gravitational, electromagnetic and weak forces.
In terms of flavour, there are six leptons – electron, muon, tau, electron neutrino, muon neutrino and tau neutrino. The first three are charged and the last three are neutral. They have their respective antiparticles.
The six leptons fall into three so-called generations or families in increasing order of mass, each consisting of a charged lepton and its neutrino. Thus, the first generation consists of the electron and the electron neutrino, the second consists of the muon and the muon neutrino, and the third consists of the tau and the tau neutrino.
Only the lowest mass first generation leptons are stable and are ingredients of common matter. The higher generations are only observed in high energy decay processes.
Quarks
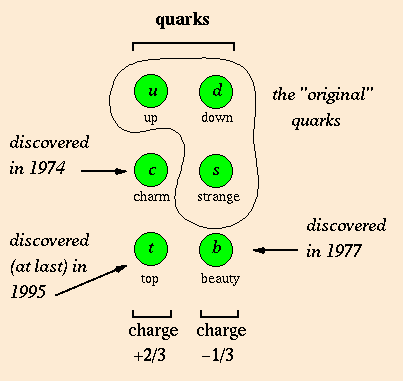
Quarks are fundamental particles that are distinguished by the presence of colour charge. As a result, they are never observed in isolation, but do interact via the strong interaction. Possessing mass, electric charge and flavour, they are also involved in the other fundamental interactions.
In terms of the mysterious flavour, there are six kinds of quarks: up, down, strange, charmed, top and bottom. Each has its own antiparticle.
Similar to leptons, quarks are three generations: the first consists of the up and down quarks, the second has the strange and charmed quarks, and the third has the top and bottom quarks. Again, in striking similarity to the leptons, the stability of quarks decreases down the generations, and only first generation quarks are found in regular matter.
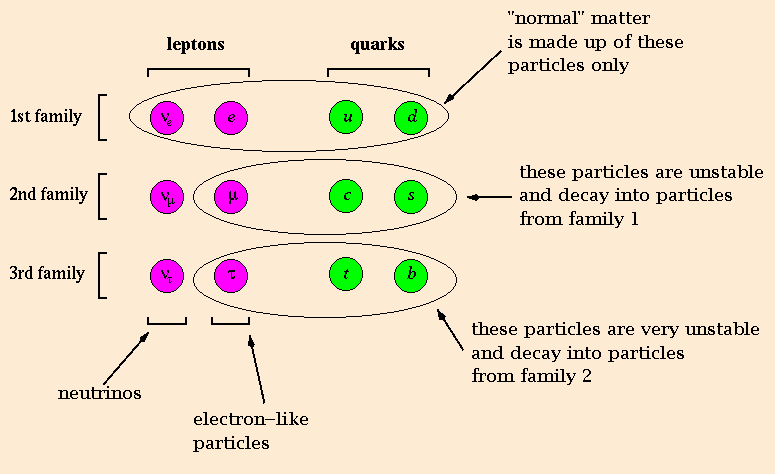
But where do we see quarks in normal matter? The answer lies in protons and neutrons. While no colour-charged particle can exist in isolation, they can exist in colourless groups. As seen in the section on the strong force, there are two simple ways of achieving this: one is to have three different colours (or anticolours); another is to have a colour and its anticolour. This is precisely what happens in nuclei. Protons and neutrons consist of three coloured quarks in appropriate fashion to give net zero colour charge. The force holding the nucleus together is mediated by pion, which consist of a quark and the corresponding antiquark, again with net colour zero.
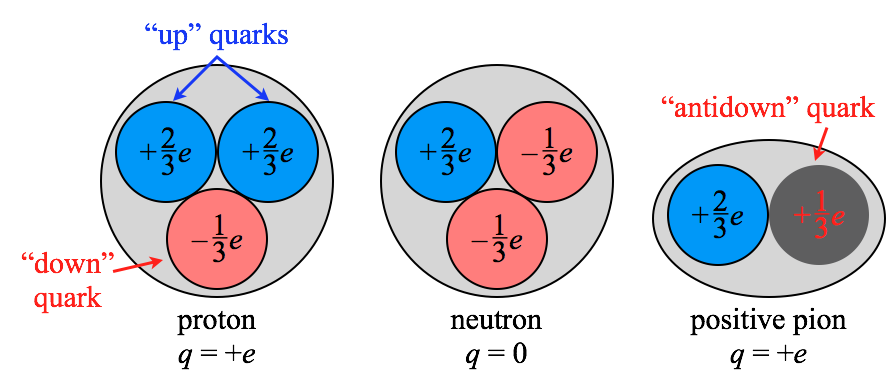
More on baryons and mesons
Baryons and mesons are not fundamental particles, but rather are collections of quarks found with zero net colour.
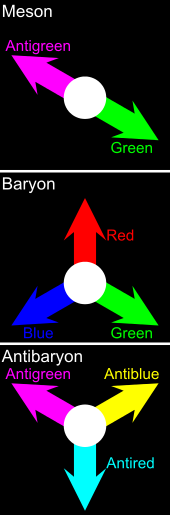
Baryons (heavy particles) consist of three quarks, arranged like in the case of the proton or neutron, to give zero colour charge. The only stable baryons consist of first generation quarks and are the proton and the neutron, found in atomic nuclei.
Mesons (middle-order mass particles) consist of two quarks, arranged like in the case of the pions seen in the case of nuclear forces. The higher the mass of the meson, the more unstable it is.
Mesons and baryons were classified by Gell-Mann, in the 1960s, by a process of arrangement called the Eightfold Way (which led to understanding of the quark theory). Put simply, they are arranged in specific geometric patterns (octuplets, decuplets, etc), based on their electric charge and strangeness (another flavour quantum number). This arrangement even led to the prediction of some undiscovered baryons, much like Mendeleev’s chemical periodic table predicting the existence of undiscovered elements.
In conclusion, the Standard Model of Physics is a largely successful attempt to explain the entire universe in terms of a fixed set of particles and their interactions. While the number of particles is quite large, it is astonishing that all the universe’s near-infinite variety and phenomena can be summed in such a neat fashion with a limited number of particles.
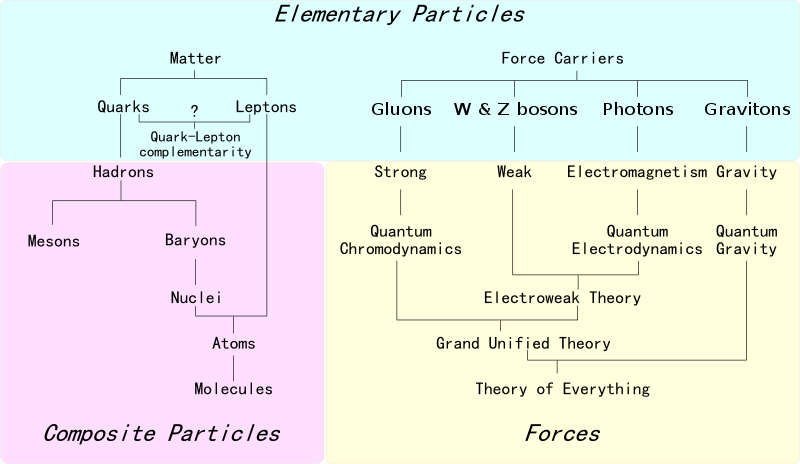
Is this the end of the road? Not quite. There are still holes that need filling, and obtaining solutions to them would make the standard model more complete. Then we would truly be able to explain the entire universe with just this set of particles and their interactions. A quest which many scientists have dedicated their lives to, and not reaped the rewards they dreamt of. Yet, the challenge is carried on, because arriving at the solution would mean we would truly have a “Theory of Everything”.
Well done samudra, all credit goes to your parents for making a genius like you. Wishing that you succeed in unravelling many mysteries in the world- Dr Ravishankar
Namaste Acharya samudra sir??..
You done a Great job sir..superb article??
I’m Lakshmi from Bengaluru. I’m a teacher, your mother Krishnapriya ma’am is my favourite great teacher.I got fortunately in WhatsApp harry potter group. Both of u blessed to have a such like a parent and a such a son. I’m not a science teacher but after reading it I got valuable and most important information. Thanks u so much sir..wishing u all the best for your great steps