Gravitational waves are one of the predictions of Einstein’s General Theory of Relativity, back in 1915. It took around a century, till 2015, for gravitational waves to actually be identified, and confirm the existence of one of the most elusive phenomena of physics.
Index
What are Gravitational Waves?
General Relativity and Space-Time
General relativity is a theory of gravity. It states the universe is four-dimensional, consisting of three space and one-time dimension. This four-dimensional continuum is called space-time, and can be modelled by a mathematical idea called “Minkowski space-time“.
The mathematics behind it is quite rigorous and shows results that are different from our usual understanding of three-dimensional space.
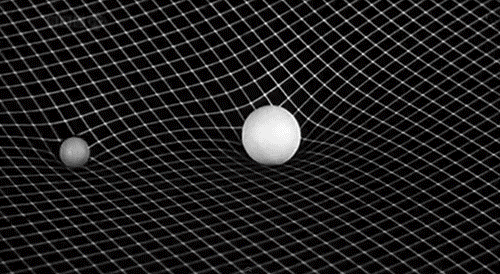
The important takeaway, as far as gravity is concerned, is this: the force of gravity is simply an effect of the bending of space-time by the mass of an object. This can be visualized in the form of a large ball making a hollow in a stretched fabric, and “pulling” other balls placed on the fabric towards itself. Thus, we often see space-time being compared to fabric.
Where does this relate to gravitational waves?
Gravitational Waves
We know that objects with mass cause distortions in space-time. What happens when these objects move?
Gravitational waves are “ripples” in space-time produced by moving objects with mass. They move at the speed of light. Technically, all objects with mass-produce gravitational waves, provided they accelerate, and do not show perfectly symmetric rotational motion. However, the gravitational force is so weak that it takes enormously large gravitational potentials, hence masses, for the waves to be detectable.
Some such systems which produce relatively large gravitational waves are orbiting neutron stars, white dwarves, and black holes.
Effects
As gravitational waves are ripples in space-time, they distort the local sense of space and time, similar to how ripples in water distort the local positioning of water. Also, since waves are periodic, these disturbances of space and time are also periodic, for a local observer.
A simple way to visualize this is to think of a ring of particles. If aligned correctly, a gravitational wave passing through them will distort the space-time such that the circle stretches upwards and sideways alternatively. The stretching could also happen at an angle, depending on a phenomenon called “polarisation” of the gravitational wave.
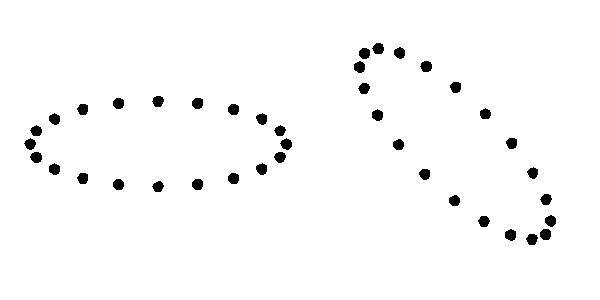
However, this stretching effect is minute. For a gravitational wave passing through earth, the stretching is around a thousand times smaller than the size of an atom. This is so small, only the most sensitive equipment can sense it.
Another effect of gravitational waves is the loss of energy. As we have seen before, orbiting objects produce gravitational waves. These waves carry away energy and make the orbiting bodies get closer, till they eventually collide or fuse. Again, the energy radiated is so small, it escapes human detection most of the time.
History and Detection
Theoretical Foundations
Even before Einstein’s theory of general relativity, other scientists had suggested gravitational waves as a possibility, based on the similarities of the laws of gravitation and electromagnetism. Scientists who worked on such ideas included Henri Poincaré and Oliver Heaviside. However, they gained prominence with Einstein’s publishing of General Relativity (GR) in 1915.
Despite the theoretical reason for their existence, most scientists at that time, including Einstein, were doubtful about their existence. Indeed, some managed to initially show that the waves were only a result of the mathematical methods used in the equations of GR, and not real physical occurrences.
It was in the late 1950s that the theoretical evidence for gravitational waves gained strength. Physicists managed to show that gravitational waves were a real prediction of GR, not a mathematical fossil. Furthermore, an interesting thought experiment by Feynman showed that gravitational waves actually carry energy, giving them more credence.
Early Experimental Endeavors
In the 1960s and 70s, scientists began looking for direct evidence for gravitational waves. The early attempts were too ambitious and often gave no results or false positives. With direct evidence failing, scientists tried to find indirect evidence. In this, the first step forward was taken in 1974 by Russell Alan Hulse and Joseph Hooton Taylor, Jr., who analyzed an orbiting binary system of pulsars, a type of neutron stars.
Their observations showed that the orbit of the binary system was shrinking. This matched predictions because gravitational waves should be carrying away the energy of the system and making the orbit get smaller.

For their work in obtaining indirect evidence for the existence of gravitational waves, Hulse and Taylor were awarded the Nobel Prize in 1993.
Towards Direct Detection: Laser Interferometry
While this indirect evidence was nice to have, scientists were curious to have direct proof as well. The experimental ideas for this were conceived as early as the 1960s and 70s, but putting them into action took longer. This was partly due to technical as well as economic constraints.
The general idea behind all the experimental apparatus was a technique called laser interferometry.
In this process, a laser beam is split into two beams, allowed to travel great distances before recombining. The recombination produces distinct interference patterns. As gravitational waves are expected to cause distortions of space-time, the distances traveled by the beams changes, resulting in noticeable changes in the interference patterns. These would provide confirmation of gravitational waves.
LIGO: Laser Interferometer Gravitational-Wave Observatory
The first successful identification gravitational waves occurred in 2015 with LIGO, an observatory consisting of two separate sets of equipment located far apart in the USA. Despite the idea being conceived in the 1980s and observations starting from 2002, the results were obtained only in 2015, when the technology was sufficiently advanced.
How does LIGO work?
LIGO is in principle a laser interferometer, as described in the previous section. However, given the weakness of gravitational waves, the set-up had to be huge enough and sensitive enough to detect the most minute results.
For this, each individual interferometer consisted of a 4 km long L-shaped tunnel, in which the laser beams travelled distances over a 1000 km by multiple reflections. Then, the sensors are sensitive enough to detect a change less than ten thousand times smaller than a proton’s width. For comparison, if the whole distance to the nearest star to the Solar system was used as a reference, the change was less than a hair’s thickness.
Two observatories were used, far apart, so that meaningless noise could be filtered out and only the actual signal would be recorded.
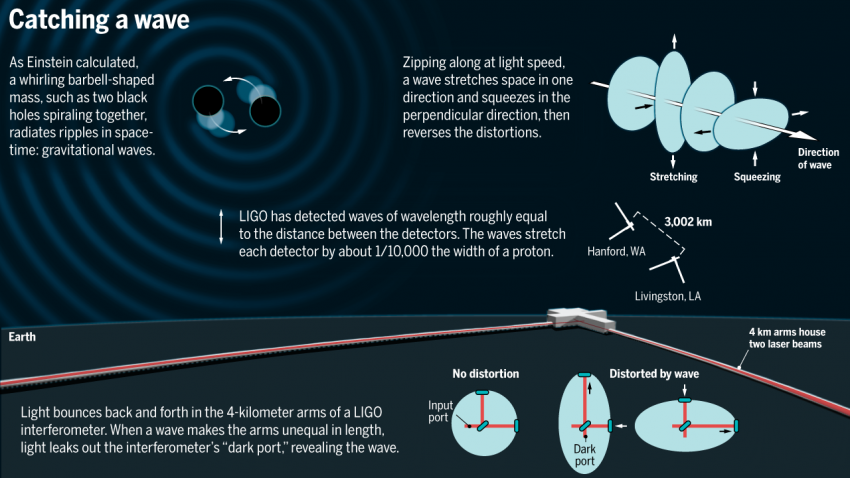
The successes
After over a decade of null results, LIGO obtained its first success in 2015. It observed the fusion of a pair of black holes, which first orbited each other before merging.
While the black holes themselves merged 1.3 billion years ago, it took so long for the waves from them to reach us and be detected. The gravitational wave carried away the mass lost by the black holes in the form of energy.
This was followed by further detection of black hole mergers in 2016 and 2017. In an even more startling step forwards, LIGO observed the merger of two neutron stars, which are visible, unlike black holes, in 2017.
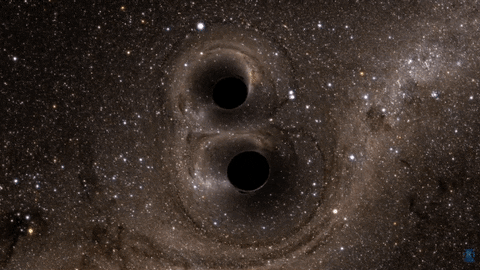
The scientific community gave LIGO its due recognition. It, along with contributors, received the Special Breakthrough Prize Award in Fundamental Physics in 2016. The scientists who were part of its journey, Rainer Weiss, Kip Thorne and Barry Barish, received the Nobel Prize in Physics in 2017.
Implications and Future Scope
The detection of gravitational waves provides evidence in favour of Einstein’s General Theory of Relativity. It also means that scientists now have a way of studying the realm of strong gravity – where gravitational potential is very high. So far, this was a theoretical area, but now experimental opportunities are opening up as well.
The fact that we can observe gravitational waves now means that we can enter a new area of astronomy: gravitational wave astronomy. Unlike traditional astronomy, which uses electromagnetic radiation (such as light, X-rays and radio waves), this form of astronomy uses gravitational waves to study celestial bodies. It is advantageous as gravitational waves barely interact with most matter, and so signals reach us unimpeded. Further, it can provide evidence of those bodies which cannot be observed with the electromagnetic spectrum: such as black holes. This also applies to the early universe, where electromagnetic observation is near-impossible because the early universe was opaque to electromagnetic radiation.
The scope ahead is vast, as this is a field in its infancy. With technological and theoretical developments, it is hoped that gravitational waves play a more important part in the understanding of the universe, and help us unravel more of its mysteries.
FAQ
Gravitational waves are “ripples” in space-time produced by moving objects with mass. These waves move at the speed of light. Technically, all objects with mass-produce these waves, provided they accelerate, and do not show perfectly symmetric rotational motion.
On earth, you can not feel gravitational waves. Interestingly, you can’t feel these waves even if two black holes collided near our sun. This is because gravitational waves dissipate very fast.