Relativity (also known as the theory of relativity) is, in the simplest form, a physical theory that states that all physical laws are the same all over the universe. Starting from this simple assumption, Einstein proceeded to derive two theories of relativity: first, the Special Theory of Relativity in 1905, and the General Theory of Relativity, in 1915. Both revolutionized the modern understanding of physics, shaking the very foundations of physics. To understand what they are, let us start with the origins of the first theory of relativity, special relativity.
Index
Origins of Special Relativity
Rest and Motion
Prior to special relativity, the prevalent theory that explained motion was that of Newton. Newton’s three laws of motion were believed to be sufficient to explain all forms of motion, in terms of forces and acceleration. It was also hugely successful when it came to experimental evidence at that time, as the results obtained were quite in agreement with the theory.
Inside the mathematical framework of Newton’s laws of motion was the Galilean transformation, which was basically a mathematical way to relate velocities in different frames of reference.

Now comes the question: what is a frame of reference? In a non-technical manner, a frame of reference is a viewpoint from which an observer makes observations. For example, the platform in a railway station is a frame of reference. The people on the platform make observations regarding the rest and motion of objects with respect to this platform.
Similarly, for people on a train moving through the platform, the train itself is a reference frame.
The basic idea of the Galilean transformation is that velocities add up across reference frames in the manner that seems to be “common sense”. To visualize that, think of a train moving at 40 km/h northwards. On the train, a person A is walking north at a speed of 5 km/h. Clearly, in our experience, to a person B on the ground, person A seems to be moving at 45 km/h northwards, because his/her 5 km/h add up to the 40 km/h of the train.
In the opposite case, consider that the person A is moving south, on the train, at 5 km/h again. To person B on the ground, it will look like A is still going north, but at 35 km/h, because their velocity of 5 km/h is opposite in direction to the 40 km/h of the train.
All this seems “common sense”, but contradictions were soon to turn up.
The Speed of Light
By the late 19th century, thanks to the work of Maxwell, Hertz, and others it was accepted that light was an electromagnetic wave that traveled at a measurable, high speed of nearly 300,000 kilometers per second. The four simple mathematical formulas involved in this theory of electromagnetism were called Maxwell’s Equations. They were expected to be universally applicable.
Interestingly, when Galilean Transformations (which seem correct by “common sense”) were applied to Maxwell’s Equations, the results caused problems. Maxwell’s equations lost their simple appearance when this happened, which meant that there was only one form in which they were simple. This led to three possibilities, at least:
- Only Maxwell’s Equations were incorrect, as they were not universally the same.
- Only Galilean Transformations were incorrect, because they altered the math of Maxwell’s transformations.
- Both were correct, but there was only one fixed reference frame in which Maxwell’s Equations were simple.
The first two possibilities felt too radical for scientists to accept then. Hence they chose to accept the third one, which meant that there was one fixed frame in which Maxwell’s Equations were simple. This frame was called the ether frame and scientists began to search after it.
The Search for Ether
The ether frame was important because it was the only frame in which Maxwell’s Equations retained their simple form. A direct conclusion, guided by the Galilean transformation, was that the speed of light is 300,000 km/s only in this frame. In any other frame, the speed of the frame should add up to the speed of light, just like in the case of the person and the train in the previous example.
There was however little or no proof for the existence of ether. Its supposed properties were also very unphysical. It was supposed to be spread throughout the universe, be extremely light, yet extremely strong, and completely invisible and exerting no influence on any physical object.
An ingenious experiment was designed at the time to test the existence of ether. It was called the Michelson-Morley experiment after the two major scientists working on it. It aimed to calculate the speed of the earth through the ether, based upon on the Galilean idea of how velocities add up.
The experiment was set up rigorously with enough accuracy to pinpoint a non-zero velocity of the earth to a high degree of precision. However, the results showed that there was no such velocity: the earth did not move with respect to the ether, if the ether even existed.

Further repetitions of this experiment and others showed that the ether theory was wrong: there was no ether in the universe and no ether frame.
Special Relativity
New Beginnings
With the ether theory discarded, new explanations had to be found for the contradictions between Galilean transformations and Maxwell’s Equations. The step forward was taken by Albert Einstein, who took a radical assumption and declared that Galilean Transformations were wrong.
This seemed to go against common sense, which strongly supported Galilean transformations. Yet, Einstein started off on his new theory with just two basic assumptions.
Postulates of Special Relativity
- All non-accelerating frames are equivalent.
- The speed of light is the same (300,000 km/s or 3 • 108 m s-1) in all reference frames.

While these may not look too drastic, they go directly against the Galilean transformation. While the Galilean Transformation implies that there is only one frame in which the speed of light is 3 • 108 m s-1, Einstein’s postulates say that the speed of light is the same in every frame.
If that does not sound unusual, think of the previous example of two people and the train. Person A’s speed, on the train, is 5 km/h, but is 45 (or 35, depending on direction) to person B on the ground. If light behaved like Person A, its speed would be 300,000 km/h on the train and 300,040 km/h to the person B on the ground.
Yet, according to Einstein, the speed of light is the same – 300,000 km/h to both people on the train and people on the ground.
The Implications
Starting from this remarkable hypothesis, Einstein proceeded to derive many results, many of which seemed to go against common sense. The general theme throughout was that all measurements are relative, and there is no absolute “correctness” of observations. Observations are correct only in whichever reference frame they are made, and do not need to agree with observations in other frames.
The fundamental effects of special relativity include length contraction, time dilation, and loss of simultaneity. In simple terms, they imply that observers in different reference frames do not, in general, on observations of length, time, and simultaneity (i.e. do two events occur at the same time?). For example, to a stationary observer, a moving object appears shorter than it does to an observer moving along with it. Another result is that time goes slower for faster moving observers.
'Time is an illusion.'― Albert Einstein Click To Tweet
Going further from these basic results, more dramatic results, often stated as paradoxes were derived. One of the most popular is the Twin Paradox, dealing with the ages of two twins, one who goes on interplanetary travel, and one who stays on earth.
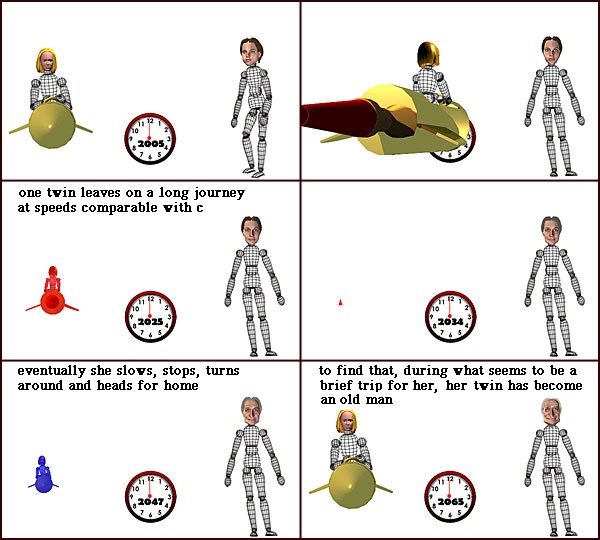
Despite the so-called paradoxes seeming nonsensical, they could be explained with careful attention to the theory.
Still, a question remains: Was there enough experimental evidence to support the then “unusual” claims of special relativity? The answer was a resounding yes.
Evidence
Evidence is strongly in favor of Einstein’s Special Theory of Relativity. The Michelson-Morley experiment, and its associated experiments, which disproved the existence of ether, were already in favor of it. Furthermore, the field of particle physics provided a variety of experiments that backed up special relativity. The measurements of mass, energy, momentum, and half-lives of particles close to the speed of light gave overwhelming evidence supporting special relativity.
Towards General Relativity
As we have seen so far, special relativity deals with non-accelerating frames of reference. With the completion of this theory, Einstein wanted to proceed to a more general theory that described motion of all forms, accelerated or not. Further, he hoped to include gravity in such a framework that could successfully explain all motion.
The Equivalence Principle
The initial steps in this process involved the Equivalence Principle: gravitational and inertial masses are equivalent. In more elaborate fashion, we can say that a person in a gravitational field (such as near the Earth) cannot distinguish their experiences from a person in an accelerating frame of reference (such as a rocket accelerating with the acceleration equal to that caused by gravity).
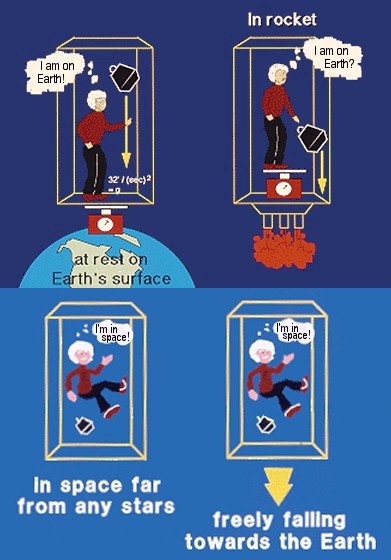
Applying these ideas to those obtained from special relativity, Einstein arrived at results that showed that mass and gravity produced relativistic effects, such as gravitational time dilation and bending of light.
Using the Mathematical Tools
At this point, Einstein proceeded to use mathematical tools such as tensors and Riemannian geometry that helped him arrive at a more complete theory of relativity, termed the General Theory of Relativity. The details are quite complicated and are beyond the scope of a simple article, but it suffices to say here that they helped understand gravity in a relativistic sense.
General Relativity
The Overview
General relativity is fundamentally a theory of gravity. Unlike Newton’s theory of gravitation, it does not treat gravity as a force, rather as a geometric property of space-time itself. It says that the presence of mass curves space-time in such a fashion that results in what we perceive as the “force” of gravity.
A common metaphor for this involves placing a large ball on a stretched rubber sheet. The sheet curves around the ball, and if another ball is placed somewhere on the rubber sheet, it moves towards the large ball as though it was “attracted” to it by a force.

General relativity also predicts many testable phenomena such as gravitational time dilation, gravitational lensing, gravitational waves and much else. These form the backbone of experimental evidence on which general relativity stands.
Evidence
Some of the earliest experiments to test the validity of general relativity were suggested by Einstein himself. These were: the anomalous orbit of Mercury, deflection of light by the sun, and gravitational redshift of light.
Einstein himself managed to explain the long-unsolved mystery of the anomaly in Mercury’s orbit around the sun, by invoking general relativity and the curvature of space-time. The proof for gravitational light deflection was first observed during a solar eclipse in 1919 when the change in position of observable stars was a direct result of general relativity.
Finally, experiments in the 1950s involving time dilation helped confirm gravitational redshifting of light too. Further, every experiment that supports special relativity also partly verifies general relativity, as the latter is a generalization of the former.
More modern experiments to verify general relativity further cemented evidence in its favour. Many cosmological observations such as gravitational lensing of light, the existence of black holes, and the orbits of the binary stars are part of this. Some of the most recent experiments include the detection of gravitational waves in 2016 and the imaging of a black hole in 2019.
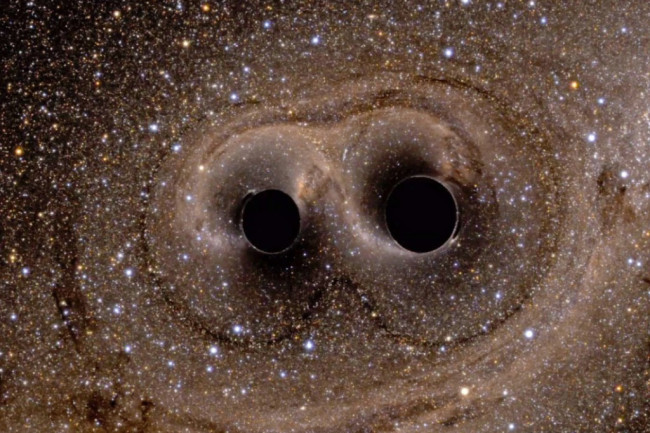
Applications of Relativity
As a complete theory of motion, relativity is applicable in all physical phenomena. However, its most explicit applications are where it diverges significantly from classical predictions. Prominently, GPS (Global Positioning System) uses both special and general relativity to account for various effects such as time dilation and loss of simultaneity that cannot be explained otherwise.
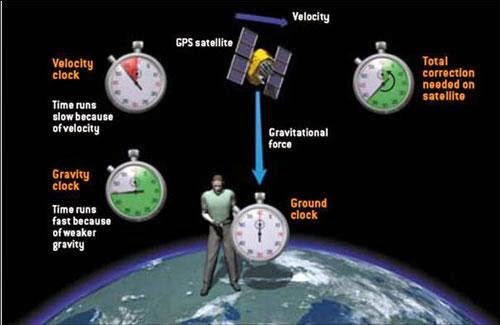
Properties of matter at speeds close to that of light are explained by relativity too. One prominent example is the color and chemical behaviour of gold, which is explained by the relativistic velocity of electrons. Relativity also helps us understand many cosmological phenomena better, such as the origin and expansion of the universe, along with the behaviour of bodies such as black holes and binary systems.
Conclusion
Relativity is a revolutionary scientific theory that reshaped our understanding of the Universe. It changed our views on what was considered basic – facts such as rest, motion, and mass. Possibly in the future, it could become part of a more unified theory in the understanding of the universe: some form of Quantum Gravity or Theory of Everything.