CRISPR-Cas is a prokaryotic (loosely bacterial in non-scientific usage) immunity system that helps bacteria defend themselves from infection by bacteriophages.
Bacteriophages are a kind of viruses that attack bacteria. CRISPR is a set of DNA sequences that help identify infecting bacteriophages. Cas9 is a protein that cuts the identified DNA at the required points. Together, they help disable invading viruses.
Index
History of CRISPR and Cas9
Terminology
DNA (deoxyribonucleic acid), the genetic material is a long stretch of building blocks called nucleotides. CRISPR are sequences of these nucleotides, found in the DNA of bacteria. CRISPR stands for Clustered Regularly Interspersed Short Palindromic Repeats. What does this mean? Let us examine:
Clustered, which refers to the fact that these sequences are bunched together.
Regularly interspersed, which refers to the fact that these sequences are not continuous, and are found in between other stretches of DNA.
Short palindromic, which means that these stretches are not very long in DNA terms, and are palindromic – they read the same back-to-front in the language of nucleotides
Repeats, which refers to the fact they are have the same pattern of nucleotides repeated.
Cas9 is a specific kind of protein, CRISPR-associated protein 9. It is part of a category of proteins, CRISPR-associated proteins (“Cas”), that help in bacterial immunity. Since Cas9 is most often used with CRISPR, the system is often called CRISPR-Cas9.
Origins in Bacteria
Bacteria are often attacked by viruses called bacteriophages (“phages”). To protect against these, a large number of bacteria developed a system called CRISPR-Cas.
When bacteria are infected by bacteriophages, the system takes up part of the bacteriophage DNA and adds it to the bacterial DNA. This added DNA is what becomes CRISPR. This way, the bacteria accumulate a library of DNA. This contains snippets of bacteriophages that tried to attack the bacteria in the past.
If the bacteria is later attacked by any phage, Cas9 uses CRISPR in the bacterial DNA to try and identify the phage. If it had already infected the bacteria before, identification is successful, and the Cas9 protein cuts the phage DNA exactly where the CRISPR directs it to. Once this cutting is done, the phage gets deactivated and is not a threat to the bacterium. The job of directing Cas9 is done by RNA (another nucleic acid found in cells).
If the phage is new, there would be no previous record of it in the CRISPR. So, the proteins associated with CRISPR (Cas1 and Cas2) help collect part of this new phage DNA for future reference, and add it to CRISPR.
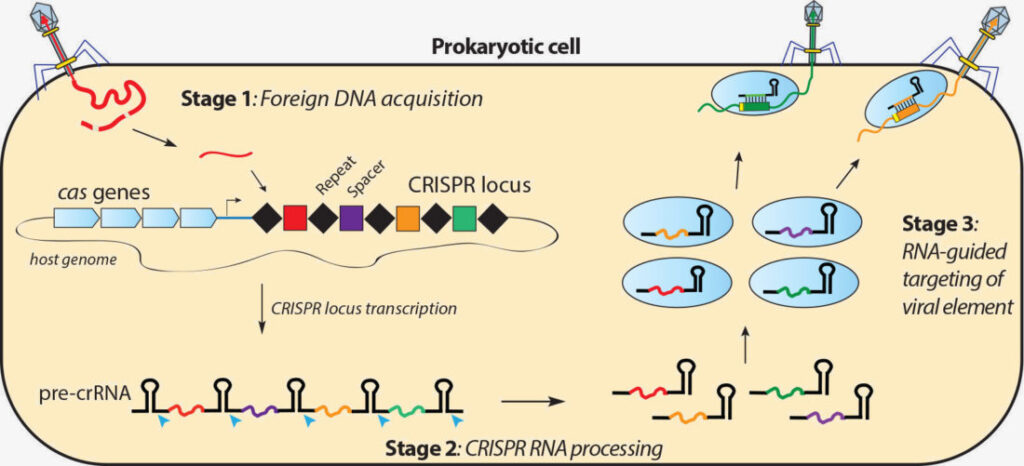
Thus, the CRISPR-Cas9 system works as some form of identification and elimination of phages in bacteria.
Discovery by Humans
Scientists discovered the DNA sequences that came to be known as CRISPR as early as in the 1980s. However, their purpose was uncertain. Research by scientists such as Francisco Mojica and Ruud Jansen showed that there were proteins (the Cas series of proteins) that were associated with CRISPR. Many scientists began wondering what function the proteins and CRISPR could serve.
Experiments in the late 2000s showed that the CRISPR-Cas system was used in bacterial immunity by disabling the infecting bacteriophage. The mechanisms involved got understood better with more research in the process.
It was around this time that scientists began to realize that the CRISPR-Cas9 system could be used in gene editing.
CRISPR-Cas9 in Gene Editing
The Concept
In naturally occurring CRISPR, the sequences help the Cas9 identify specific parts of phage DNA and cut it there. Scientists Emmanuelle Charpentier and Jennifer Doudna modified this natural system to get a new, artificial version that could identify any specific DNA of the experimenter’s choice. All that was needed was RNA that matched (in science terms: was complementary to) the DNA that needed to be cut. This guide RNA helped the Cas9 find the exact required DNA.
Because of this, CRISPR-Cas9 became a very specific, wide-ranging tool for gene editing. Any known sequence in DNA could be targeted and cut as needed.
Once the idea of artificial CRISPR-Cas9 in gene editing took shape, many scientists extended to different species of living organisms. Thus, they were able to perform genetic editing in various species.
How Does The Process Work?
DNA consists of two strands of nucleotide chains. As we have already seen, CRISPR-Cas9 system makes a break in the targeted DNA, and breaks both strands in what is called a Double-Stranded Break (DSB). The cell now tries to repair the DSB, and this is where the genetic modification can happen.
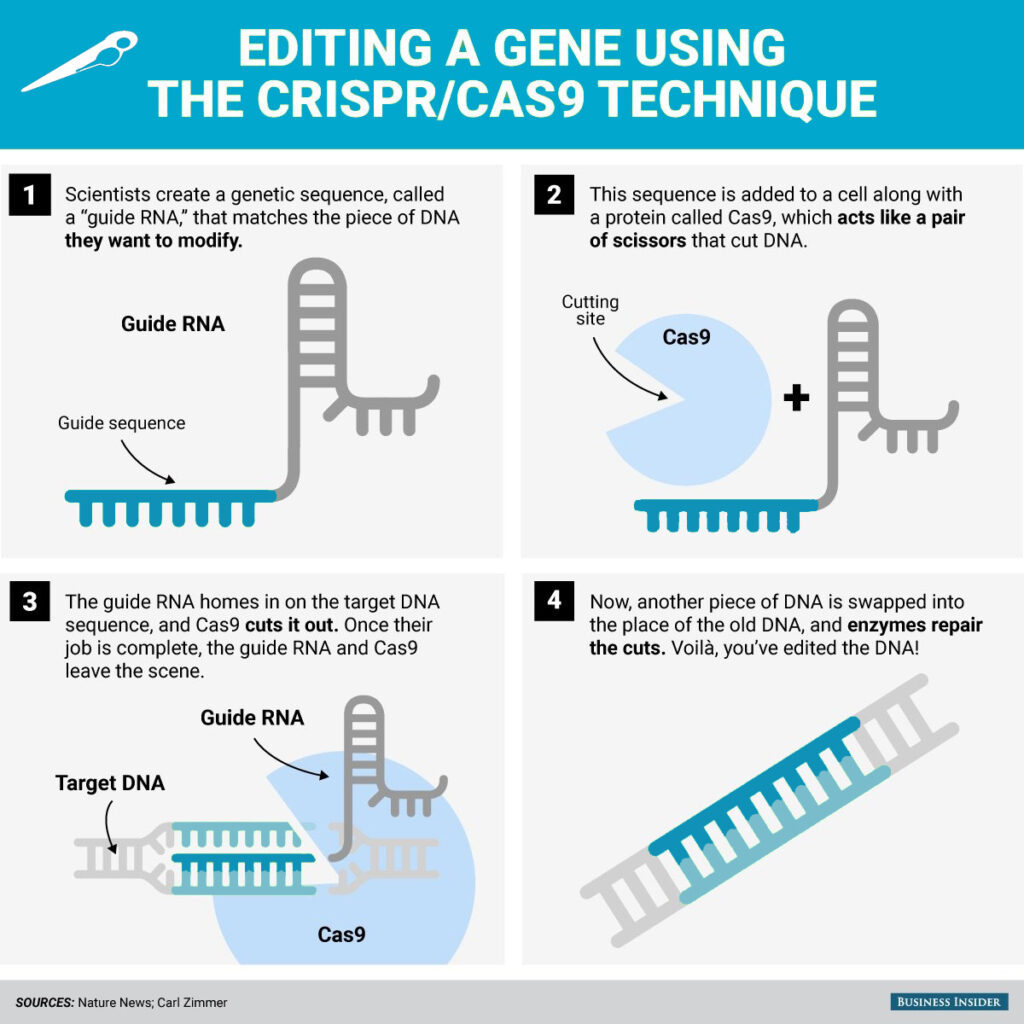
Two major mechanisms involved in DSB repair. One is Homology-Driven Repair, the other is Non-Homologous End Joining.
- In Homology Driven Repair (HDR), the cell attempts to use other DNA in the cell as a template to repair the broken strands. In the artificial use of CRISPR-Cas9, this template is replaced by a chosen piece of DNA that the scientists want to insert. As the repair occurs, the chosen DNA is inserted. Thus, gene editing is done.
- In Non-homologous End Joining (NHEJ), the cell simply attempts to ‘glue’ the broken ends together. While this system is faster, it is more error-prone than HDR. Scientists use the errors that occur in the ‘gluing’ process to ‘switch off’ or ‘knock out’ specific genes. Thus, they can understand the importance of the gene by seeing how the cell behaves when it is switched off.
Applications
DNA, the genetic material, is the molecule that controls processes in living organisms. By being able to control it, scientists have a great amount of control over what occurs in these processes.
Curing Diseases
Some problematic sequences in DNA are behind inherited diseases. Being able to edit DNA could mean permanently fixing these diseases, by eliminating the problematic sequences. CRISPR-Cas9 is very helpful in this regard because it can help focus on the very specific problem sequence.
For example, there are procedures under trial to help fight certain forms of cancer and blood diseases. CRISPR-Cas9 was used in 2017 to correct a mutation leading to heart disease in an embryo. Research is underway regarding other treating inherited diseases too, such Huntington’s Disease, that affects the brain and HIV-AIDS.
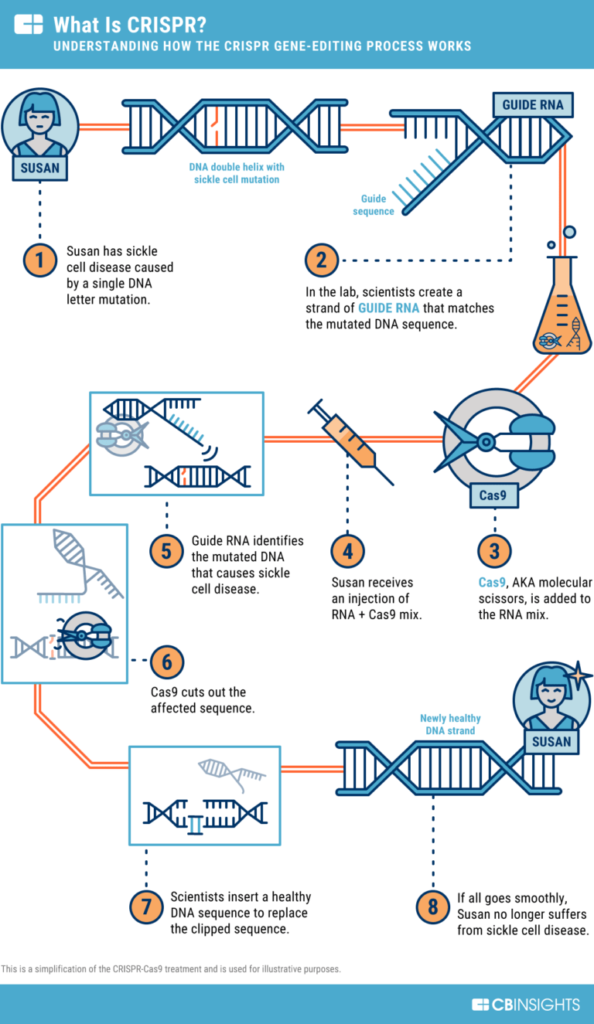
Improving Crops
CRISPR-Cas9 can be used to edit genes of crops to obtain specific desired traits in them. For example, scientists in the US have incorporated genes in white mushrooms that slows them down from turning brown and remaining viable for longer.
Some forms of algae have been edited to have greater biofuel content. Other crops have been edited to enable them to have greater yield or nutrient content. Unlike transgenic GMOs (Genetically Modified Organisms), this does not involve using genes of other species and could be safer, and more acceptable for the public.
Research
As CRISPR can be used to target and cut genes at very specific points, it can help us understand those genes better. By deactivating the genes, we can see what the impact of them is on the cell. Thus, we can understand the function of each gene.
Further, CRISPR-Cas9 can be used to make animals models to study human diseases and understand more about them. This could potentially lead us to discovering a cure.
Editing the human genome?
With the versatility of CRISPR-Cas9 at out disposal, it could potentially be used to edit the human genome itself to give desired outcomes. This goes beyond simply curing disease as shown before. It could lead us down a path of choosing certain traits as more desirable and selectively passing them on to future offspring.
The non-scientific term for this would be “designer babies“, referring to the fact that it seems possible to have whatever traits we chose in the babies.
However, this idea raises many concerns, which will be addressed in the next section.
Concerns about CRISPR-Cas9
While CRISPR-Cas9 is a powerful gene-editing tool, it raises many ethical and health-related concerns. For one, it is much easier to access and use, when compared to other gene-editing techniques. This means that it could be used unauthorizedly by people in various fields for their own purposes.
Another issue is unintended side-effects. Using CRISPR to edit one part of the genome might accidentally cause effects elsewhere that we do not notice. The results could turn up much later and cause harm that cannot be avoided.
Gene drive is another risk. This refers to the process where genes spread along with populations faster than they normally would, as a result of editing. As many species could potentially be edited by CRISPR-Cas9, this could result in unexpected/unwanted genes turning up in large numbers in the wild populations of the experimented species.
The issue of designer babies is also thorny. If some traits, such as ‘higher intelligence’ or ‘beauty’ are valued over others, the others could systematically be overwritten in the human genome. This could lead to eugenics. Further, with money involved, only the rich might have access to such services, which would have a profound societal impact.
Unauthorized testing could also pose many health hazards, especially for uninformed volunteers. While there is a ban on most forms of usage of CRISPR technology on humans, this is enforced loosely in some countries. As a result, we see instances such as one in 2018 when CRISPR was used to edit the genome of twin embryos in China. While the children were healthy at birth, risks for their future health are unknown. This is a case of reckless risking of human life.
Conclusions

CRISPR-Cas9, and the CRISPR-Cas system in general, is a powerful one that is changing the face of modern gene technology. It has potential to revolutionize many fields and radically change the arena of genetics. With such promise, it could be used in many beneficial ways for humanity, and the world at large.
With great power, however, comes great responsibility. A tool this powerful needs to monitored well and ethical issues addressed so it does not fall prey to misuse and hurt us in the long run.